Easy as GCxGC
Is there a place for two-dimensional gas chromatography in cannabis testing?
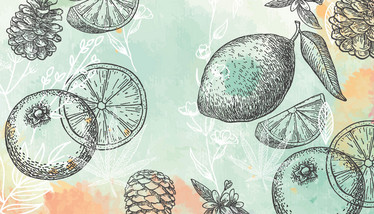
The need for robust methods of analyzing cannabis constituents continues to grow, and analytical chemists now have a wide array of techniques at their fingertips. One of the exciting things about cannabis testing is the opportunity it offers to stretch the limits of the available technology. Whether it’s automating sample preparation to ensure homogeneity of these complex (and highly variable) matrices, or helping cannabis growers to get to grips with the testing of their products, researchers and manufacturers face a number of challenges when it comes to pinning down exactly what’s in a cannabis sample, in order to provide the raw data that enables assessment of safety and efficacy. However, one group of cannabis compounds in particular – the terpenes – remain highly challenging, despite all these advances. Could two-dimensional gas chromatography shake off its ‘academic’ aura and offer a solution to this difficult but important group of cannabis compounds?
The terpene challenge
Over 100 terpenes are known to occur in cannabis and are important not just because of their distinctive aromas and flavors, but also because of their contributions to psychoactive effects such as sedation and anxiety relief (Figure 1) (1,2). In addition, terpenes also display what’s known as the ‘entourage effect’ (5,6) – the greater therapeutic effect resulting from the presence of terpenes alongside psychoactive cannabinoids. Kevin Koby, Chief Scientific Officer of Abstrax Tech, a leading provider of terpene blends to the cannabis industry, said, “Because of the entourage effect, an understanding of the terpenoids in a cannabis product can be really important to growers wanting to ensure consistent effects from their products. For example, high myrcene content is associated with the sedative effect known as ‘couch lock,’ whereas elevated levels of pinene are associated with alertness.”
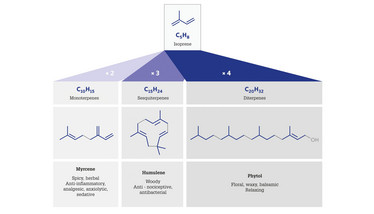
Figure 1. The classification of terpenes according to the number of isoprene units they contain in their molecular skeletons, showing examples of three compounds found in cannabis, with their aroma (3) and effects on the human body (2,4).
The consequence of this, he says, is that plant breeders will often attempt to engineer cultivars with specific terpene profiles, in order to encourage particular traits (7). However, this relies on in-depth terpene testing to allow the best cultivars to be selected – and that’s a big challenge.
So what makes terpenes so tricky to analyze? It’s largely down to their immense variety. Not only can the fundamental carbon backbones take a variety of shapes, but there are myriad derived forms (terpenoids) to contend with, as well as isomers with very similar properties. As a result, separating all these from each other – let alone confidently identifying them – is enough of a challenge on its own.
Taking on terpenes
Deciding how best to separate and identify terpenes in cannabis used to be a major preoccupation for many analysts – and continues to be a point of discussion (8). This is primarily because there are no defined regulatory methods for cannabis testing, meaning that the decision on which technique to use lies largely with the analyst. Methods available include high-performance liquid chromatography (HPLC) or gas chromatography (GC), with the detection method depending upon factors including detection limit, and analyte volatility and stability.
However, for relatively volatile species such as terpenes and residual solvents, GC is the clear winner for its general applicability and ease of use. It is usually coupled with headspace sampling, which is inexpensive, highly effective and easily automated, with the added advantage that it removes the need for labor-intensive solvent extractions.
Unfortunately, choosing a detection method for GC analysis of cannabis terpenes is less straightforward. David Mathis from Bonafides Laboratory Inc. – a cannabis testing facility based in Colorado, USA – has particular experience in this regard. He said, “Flame ionization detection is ideal for quantitation, but we often find that it over-estimates the abundance of important terpenes. Mass spectrometry and vacuum ultraviolet detection, although allowing the differentiation of co-eluting compounds, are typically more expensive […] and they also often require more experienced analysts.” An additional factor is that the algorithms used to deconvolve structurally similar terpenes can be prone to errors.
The solution to this conundrum, as Mathis sees it, is to try to improve the quality of the GC separation. “Confidence in compound identity and quantitation is primarily constrained by the degree of separation,” he says. “Being able to resolve co-elutions gives you a lot more flexibility when it comes to choice of detector.” So how can the separating power of GC be improved while retaining its undoubted practical advantages? The answer may lie in a well-established but overlooked variant of the technique – two-dimensional gas chromatography, usually abbreviated as GC×GC. First developed in the 1990s, GC×GC involves coupling two GC columns with different stationary phases, with individual portions from the first column passed into a faster-eluting second column (Figure 2). The result is separation of the sample in two dimensions (9) which provides the greater peak capacity needed to deal with highly complex mixtures like cannabis (10).
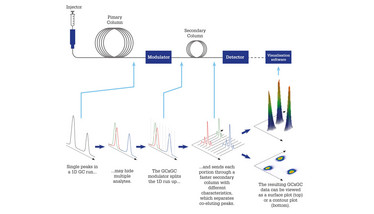
Figure 2. Schematic of a GC×GC system showing how chromatographic resolution can be improved using two columns of different selectivity.
Changing methods, changing minds
GC×GC has long been used in the petrochemical and fragrance fields precisely because of its ability to separate highly complex mixtures (11,12). For example, in the case of petrochemicals, it’s essentially the only choice if you want to have any hope of separating the sub-classes of mixtures comprising literally thousands of hydrocarbons (13). For analysis of fragranced mixtures such as essential oils, it’s the ability to separate and identify isomers that is the major advantage.
But in other fields, cannabis included, GC×GC has struggled to gain much traction. In our view, this stems from two misconceptions – complexity and cost.
GC×GC is often described as being complicated, with the impression being that it’s only used by academics for niche applications. In fairness, running GC×GC methods is more involved than for regular GC, with additional method parameters to optimize and a different format for visualizing results… but it’s worth noting that early adopters of the technology were happy to have it seen as an “advanced technique” in order to elevate the status of their research! However, the unwanted side-effect of this has been that the “complicated” label has stuck, meaning that it has often been overlooked for routine applications.
With regard to the second point, high running costs are considered to be a drawback of GC×GC systems, mostly due to the requirement for liquid cryogen by the modulator. This critical part of the GC×GC system ensures that peaks eluting from the first column are sampled and re-injected as narrow chromatographic bands into the short secondary column, to avoid overloading it and to preserve the separation achieved in the first dimension. The first commercial GC×GC modulators used liquid nitrogen to operate a so-called “cold jet,” and these thermal modulators still have a wide user base in the GC×GC community.
Beyond the misconceptions
Developments in GC×GC have now addressed both of these challenges. Numerous commercial packages are now available that allow existing GC systems to be upgraded easily, with tailor-made software to visualize and compare chromatograms as easily as in 1D systems. Not only that, but as the technique becomes more widely adopted, ready-optimized sets of columns and conditions are becoming available.
On the cost front, the running costs of GC×GC are lower than they used to be, thanks to the development of devices that use flow control rather than temperature control to achieve effective modulation. Such flow modulators don’t require liquid cryogen or chiller units, lowering both capital and running costs, and making GC×GC more affordable for small labs.
These changes have recently resulted in a greater uptake of GC×GC by high-throughput labs working on routine applications, particularly in flavor and fragrance analysis and environmental investigations. The reasoning has been that because GC×GC is capable of handling relatively complex sample matrices, it’s not necessary to spend so much time on sample preparation, which up until now has been a major drain on laboratory resources.
Not only that, but in some cases, new legislation is driving the need for advanced analytical techniques like GC×GC. For example, the Canadian Ministry of the Environment has now listed GC×GC as an acceptable analytical technique, and have published regulatory methods on using it (14).
A single method
So what can GC×GC do for cannabis terpenes? For a start, it enables monoterpenes and sesquiterpenes to be easily distinguished, because they elute in well-separated bands when using a non-polar 1D column and a polar 2D column (the so-called “normal-phase” setup). Furthermore, using this configuration means that oxygenated terpenes elute later in the (more polar) second dimension. This structured ordering simplifies the identification process, allowing tentative identifications to be assigned based on groupings alone (Figure 3).
The improved separation of GC×GC also allows common co-elutions to be resolved using simple, inexpensive detectors (such as flame ionization detection) rather than resorting to mass spectrometry or complicated deconvolution algorithms. This means that cannabis producers can obtain more reliable information on the component terpenes, which in turn allows the creation of more precise descriptions of aroma characteristics and therapeutic traits for each cultivar (Figure 4).
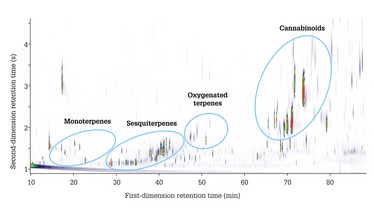
Figure 3. GC×GC color plot of a cannabis extract, showing separation of analytes according to chemical class (15).

Figure 4. The GC×GC separation of a mix of terpenes commonly found in cannabis shows that even in a clean, simple standard there are components that would co-elute in 1D GC.
In addition, more terpenes can be monitored in a single method. For example, the difficulties in separating terpenes by one-dimensional GC means that in many labs only a select group of “common” cannabis terpenes are monitored. This means that important aroma and flavor characteristics or beneficial therapeutic effects can be overlooked. Using GC×GC, cannabis producers can provide more accurate labeling of their products, providing consumers with the information that allows them to select the best product for their needs.
Thomas Martin, R&D Flavour Chemist at Abstrax, said, “Obtaining a detailed understanding of the terpenes present in cannabis products is essential both for growers and consumers alike, and GC×GC is a valuable tool for achieving this.” It has even been shown that GC×GC analysis of cannabis extracts can aid classification of samples based on the strain of plant (16). This is a very attractive prospect for plant breeders working on engineering new strains with specific therapeutic traits.
Analytical advantages
In summary, GC×GC is in the process of transforming from being a niche to a mainstream technology, thanks to advances in user-friendliness that make it far easier to employ on a routine basis. This is making it more appealing to analysts in a wide range of industries who need to achieve confident identification of a large number of volatile constituents in a wide range of matrices. Martin is optimistic about what the future holds. “With GC×GC, we now have the opportunity to answer important questions regarding why cannabis smells, tastes and affects us the way it does – and those employing it stand to gain considerable advantages in this fledgling industry.”
- EP Baron, “Medicinal properties of cannabinoids, terpenes, and flavonoids in cannabis, and benefits in migraine, headache, and pain: An update on current evidence and cannabis science”, Headache, 58, 1139–1186 (2018). DOI:10.1111/ head.13345 1139–1186 / DOI: 10.1111/head.13345.
- F Grotenhermen and E Russo (eds), “Cannabis and Cannabinoids: Pharmacology, Toxicology, and Therapeutic Potential”, Psychology Press: 2002.
- The Good Scents Company Information System (search facility), www.thegoodscentscompany.com/search2.html (accessed on 24 January 2019).
- EB Russo, “Taming THC: potential cannabis synergy and phytocannabinoidterpenoid entourage effects”, Br J of Pharmacol, 163, 1344–1364, (2011). DOI: 10.1111/j.1476-5381.2011.01238.x.
- Abstrax Tech, “What is the cannabis entourage effect (and how your brand can use it!)” (2018). Available at bit.ly/2HHw7nd.
- S Blasco-Benito et al., “Appraising the ‘entourage effect’: Antitumor action of a pure cannabinoid versus a botanical drug preparation in preclinical models of breast cancer”, Biochem Pharmacol, 157, 285–293, (2018). DOI: 10.1016/j.bcp.2018.06.025.
- CM Andre et al., “Cannabis sativa: The plant of the thousand and one molecules,” Front Plant Sci, [Epub] (2016). DOI: 10.3389/fpls.2016.00019.
- A Leghissa et al, “A review of methods for the chemical characterization of cannabis natural products”, J Sep Sci, 41, 398–415, (2018). DOI: 10.1002/jssc.201701003.
- W Bertsch, “Two-dimensional gas chromatography. Concepts, instrumentation, and applications – Part 1: Fundamentals, comprehensive two-dimensional gas chromatography”, J Sep Sci, 1999, 22: 647–665, DOI: 10.1002/(SICI)1521- 4168(19991201)22:12647::AID-JHRC647>3.0.CO;2-V.
- JB Phillips and J Beens, “Comprehensive two-dimensional gas chromatography: A hyphenated method with strong coupling between the two dimensions,” J Chromatogr A, 856, 331–347, (1999). DOI: 10.1016/S0021-9673(99)00815-8.
- L Ramos (ed), Comprehensive Two Dimensional Gas Chromatography (Comprehensive Analytical Chemistry, Volume 55), Elsevier Science, (2009).
- F Bertoncini et al., “Gas chromatography and 2D-gas chromatography for petroleum industry: The race for selectivity”, IFP Energies Nouvelles Publications: 2013.
- PJ Schoenmakers et al., “Comparison of comprehensive two-dimensional gas chromatography and gas chromatography–mass spectrometry for the characterization of complex hydrocarbon mixtures”, J Chromatogr A, 892, 29–46, (2000). DOI: 10.1016/S0021-9673(00)00744-5.
- AM Muscalu et al., “A routine accredited method for the analysis of polychlorinated biphenyls, organochlorine pesticides, chlorobenzenes and screening of other halogenated organics in soil, sediment and sludge by GCxGC-μECD”, Anal and Bioanal Chem, 401, 2403–2413, (2011). DOI: 10.1007/s00216-011-5114-0.
- SepSolve Analytical, “Improving confidence in the quantitative analysis of cannabis terpenes using flow-modulated GC×GC–FID (White Paper 014)” (2017). Available at: www.sepsolve.com/analysis-of-cannabis-terpenes/
- J Omar et al, “Resolution of co-eluting compounds of Cannabis sativa in comprehensive two-dimensional gas chromatography/mass spectrometry detection with multivariate curve resolution-alternating least squares”, 121, 273–280, (2014). DOI: 10.1016/j.talanta.2013.12.044.
SepSolve Analytical Ltd, Peterborough, UK
SepSolve Analytical Ltd, Peterborough, UK